Near-eye light field display
- See also: Terms and Technical Terms
Introduction
- See also: Near-eye display, Lightfield and Vergence-accommodation conflict
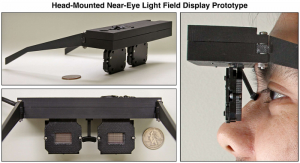
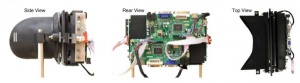
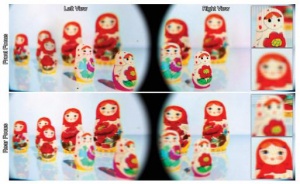
A Near-eye lightfield display (NELFD) is a type of Near-eye display (NED), often implemented in a Head-mounted display (HMD), designed to reproduce a lightfield, the complete set of light rays filling a region of space, rather than just a single flat image for the viewer. The concept of the light field, representing light rays at every point traveling in every direction (often described as a 4D function), emerged in computer graphics and vision research in the 1990s.[1] Unlike conventional displays which typically emit light isotropically from each pixel location on a fixed plane, a light field display aims to "support the control of tightly-clustered bundles of light rays, modulating radiance as a function of position and direction across its surface."[2]
By emitting light rays with potentially correct spatial and angular distribution, a NELFD allows the viewer’s eyes to engage natural vergence and accommodation (focusing) responses simultaneously. This capability aims to resolve the vergence-accommodation conflict (VAC), a common source of visual discomfort (including visual fatigue, eye strain, and headaches) in conventional stereoscopic displays used in virtual reality (VR) and augmented reality (AR).[3][4] Resolving the VAC can lead to potentially sharper, more comfortable, and more realistic three-dimensional visual experiences, especially during extended use. As Huang et al. (2015) noted, “correct or nearly correct focus cues significantly improve stereoscopic correspondence matching, 3D shape perception becomes more veridical, and people can discriminate different depths better.”[5]
Near-eye displays confront the fundamental problem that the unaided human eye cannot easily accommodate (focus) on display panels placed in very close proximity.[2] Conventional NEDs typically use magnifying optics to create a virtual image appearing further away, but these optics can add bulk and weight.[6] Light field approaches offer an alternative path to achieving comfortable viewing, potentially in thinner and lighter form factors.
Principle of Operation
Near-eye lightfield displays function by synthesizing the light field corresponding to a virtual scene within the viewer's natural accommodation range, directly in front of the eye. This means controlling not just the intensity and color of light from each point, but crucially, the direction of the light rays entering the viewer's pupil. The goal is to accurately mimic how light rays from a real 3D scene would arrive at the eye, triggering correct focus (accommodation) and retinal defocus blur cues. This control occurs within a specific viewing volume known as the eyebox; a sufficiently large eyebox allows for some natural eye movement without losing the 3D effect or focus cues, related to the concept of the Exit pupil in optical systems.
Common technical approaches to generating the near-eye lightfield include:
- Microlens Array (MLA) based: An array of tiny lenses is placed over a high-resolution display panel (like an OLED or LCD). Each microlens covers multiple underlying pixels and projects their light in specific directions, creating slightly different views for different parts of the eye's pupil. This technique, related to integral imaging or plenoptic camera principles,[2] effectively samples the light field but inherently trades spatial resolution for angular resolution (i.e., the number of distinct views or depth cues provided).
- Multi-layer Displays: Using multiple stacked, typically transparent, display layers (for example LCDs) that multiplicatively modulate light passing through them. By computing and displaying specific patterns on each layer, often using computational display techniques, the directional light distribution of a target light field can be approximated. This approach can potentially offer more continuous focus cues over larger depth ranges compared to methods with discrete views.[5]
- Varifocal / Multifocal Displays: Using optical elements whose focal length can be changed rapidly, such as tunable lenses, Deformable mirrors, or mechanically actuated lenses/displays. These systems present images focused at different distances sequentially (time-multiplexed) or simultaneously (multifocal). The visual system integrates these rapidly presented focal planes into a perception of depth with corresponding accommodation cues, effectively approximating a lightfield effect.[7]
- Scanning / Projection: Using highly collimated light sources like lasers combined with fast scanning mirrors (such as MEMS mirrors) or specialized projection optics to directly synthesize the lightfield, drawing rays point-by-point or line-by-line towards the eye's pupil.[8]
- Holographic Approaches: Using a Spatial light modulator (SLM), typically modulating the phase of coherent light (usually from a laser), to reconstruct the optical wavefront of the desired 3D scene via diffraction. This can potentially provide highly accurate focus cues across a continuous depth range but faces significant challenges like speckle artifacts and extremely high computational requirements for real-time hologram generation.[9] Holographic optical elements (HOEs) or Metasurfaces can also be used to manipulate light directionally, often combined with a microdisplay.
- Computational Approaches: Combining specialized optics (which might not fit neatly into the above categories) with sophisticated rendering algorithms. This can include techniques like compressive light field displays, which aim to represent the light field more efficiently, or optimizing light patterns projected onto diffusers or other specialized optical elements.[10]
Advantages
Compared to conventional stereoscopic near-eye displays, NELFDs offer several potential advantages:
- Correct Focus Cues & VAC Resolution: The primary intended advantage. By reproducing directional light rays, NELFDs allow the eye's natural accommodation mechanism to work correctly for the depicted virtual scene depth, mitigating or resolving the vergence-accommodation conflict.
- Reduced Visual Fatigue: Directly related to resolving the VAC, this can lead to significantly decreased eye strain, headaches, and potentially simulator sickness associated with prolonged use.[3]
- Improved Depth Perception: Providing a richer set of depth cues (binocular disparity, vergence, accommodation, and defocus blur) leads to a more robust, realistic, and accurate perception of 3D space and shape.[5]
- Thinner/Lighter Form Factor (Potentially): Certain NELFD approaches, particularly MLA-based designs, can replace bulky conventional magnifying optics with very thin optical elements, potentially enabling HMDs closer to the form factor of standard eyeglasses.[2]
- Smoother Parallax: Can provide more continuous motion parallax as the viewer moves their eye slightly within the eyebox, enhancing the sense of realism.
- Wider Eye Box (Potentially): Some lightfield display designs might offer a larger eyebox compared to conventional NEDs that rely on projecting into a small exit pupil, increasing user comfort and tolerance to HMD positioning. However, achieving a wide eyebox often involves trade-offs with other parameters like resolution or brightness.
- Potential for Prescription Correction: The ability to precisely control light rays allows some lightfield approaches to computationally pre-distort the displayed image to correct for the viewer's specific refractive errors (like myopia or hyperopia), potentially eliminating the need for users to wear prescription glasses with the HMD.[11][2]
- See-Through Capability: Like conventional NEDs, lightfield optics can be designed for augmented or mixed reality applications, allowing virtual light fields to be overlaid onto the real world.
Challenges
Despite their promise, NELFDs face significant technical hurdles that have so far limited their widespread adoption:
- Resolution Trade-off: A fundamental challenge, especially for spatially multiplexed approaches like MLAs. There is often an inherent trade-off between spatial resolution (perceived image sharpness, pixels per degree) and angular resolution (the density of directional rays, affecting the quality of focus cues and depth range). Improving angular resolution typically comes at the cost of spatial resolution for a given microdisplay panel resolution.[2]
- Computational Complexity: Generating the complex view-dependent data required for a lightfield display (rendering potentially hundreds or thousands of unique perspectives or calculating complex holographic phase patterns) is computationally extremely intensive. It requires significant GPU power, high bandwidth, and sophisticated rendering algorithms, making real-time performance for interactive applications a major bottleneck.[9][2]
- Form Factor and Weight: While some designs aim for thinness, implementing the necessary complex optics (precisely aligned MLAs, multiple display layers, bulky SLMs, fast scanning systems, or varifocal mechanisms) within the strict size, weight, and power constraints of a comfortable wearable device remains very difficult.
- Field of View (FoV): Achieving a wide field of view (comparable to human vision or immersive VR headsets) simultaneously with high spatial resolution, high angular resolution, a large eyebox, and a compact form factor is exceptionally challenging due to optical limitations and computational costs.
- Brightness and Contrast Ratio: Many NELFD techniques involve splitting light, multiple layers, masks, or diffractive elements, which can significantly reduce light throughput, leading to lower overall brightness compared to simpler direct-view displays. Contrast can also be affected by stray light or optical crosstalk between views.
- Artifacts: Different implementations suffer from unique visual artifacts that can degrade the experience. These include speckle in coherent holographic systems,[9] visible transitions or latency in varifocal systems switching between focal planes, diffraction effects from small features (like pixels or microlenses), Moiré patterns, aliasing, and image discontinuities or blur at the edges of the eyebox.
- Calibration: Manufacturing and assembling NELFDs requires extremely high precision. Aligning microdisplays, MLAs, and other optical components with micron-level accuracy is critical. Precise calibration, often requiring computational correction, is needed to ensure correct view generation and minimize artifacts.[2]
Recent reviews continue to track research aimed at overcoming these challenges through advancements in display technology (for example MicroLED panels), new optical designs, and more efficient computational techniques.[12][13]
Historical Development and Notable Examples
While the underlying concept of capturing and displaying light fields dates back to Gabriel Lippmann's integral photography (1908)[14], focused development on *near-eye* lightfield displays intensified significantly with the resurgence of interest in VR/AR spurred by devices like the Oculus Rift and the increased understanding of the VAC problem's impact on user comfort (around 2008 onwards).[3][2]
Key research milestones and notable prototypes/products include:
- NVIDIA Near-Eye Light Field Display (2013): Presented by Lanman and Luebke (NVIDIA Research) at SIGGRAPH 2013, this influential prototype (Figure 1) used microlens arrays (Fresnel #630) placed over high-resolution OLED microdisplays (Sony ECX332A, 1280x720 per eye, 12µm pixel pitch, ~2100 ppi). It clearly demonstrated the ability to provide accurate focus cues resolving the VAC within a thin form factor (1cm eyepiece thickness). Users reported being able to focus at different depths, although perceived spatial resolution was reduced (~146x78 pixels in the ~29°x16° FoV demo configuration). The prototype also showcased software-based prescription correction and used GPU-accelerated rendering.[2][1][15]
- Stanford / NVIDIA Light Field Stereoscope (2015): A follow-up collaboration presented at SIGGRAPH 2015 (Figure 2), this HMD used two stacked LCD panels per eye, driven by non-negative light field factorization algorithms. Based on Wheatstone's stereoscope design, it aimed to provide more continuous focus cues over a larger depth range (0.2m to infinity demonstrated) compared to the MLA approach, within a ~30° FoV. The goal was a practical, potentially inexpensive technology supporting focus cues in a wearable form factor, though the prototype's effective resolution per eye (approx. 640x800) was limited.[5][4][16] NVIDIA presented a revision at the VRLA Expo in 2016, emphasizing real-time CUDA-based light field computation and the potential to reduce motion sickness.[17]
- NVIDIA / UNC Holographic HMD (2017): Researchers from NVIDIA and the University of North Carolina demonstrated a holographic near-eye display using a high-resolution (2k x 2k) phase-only SLM. It achieved real-time hologram synthesis on a GPU at 90 Hz over an 80° FoV, showcasing the potential of holography for accurate wavefront reconstruction and focus cues, while also highlighting the associated computational challenges and speckle issues.[9]
- Avegant Light Field Technology (2017 onwards): Avegant demonstrated mixed reality display prototypes based on providing multiple simultaneous focal planes (reportedly 2–3 planes) within an approximately 40° FoV, aiming to address VAC in AR.[18]
- Magic Leap One (2018): Launched as the "Creator Edition", this was the first widely marketed commercial AR HMD explicitly referencing lightfield concepts (using the term "photonic lightfield chip"). Its actual implementation relied on waveguides presenting imagery at two fixed focal planes (approximately 0.5m and infinity), offering a limited form of multifocal display rather than a full lightfield, over a diagonal FoV of about 50°.[19]
- Meta Reality Labs Research Half-Dome Series (2018-2020): Meta (formerly Facebook) showcased a series of advanced varifocal VR research prototypes. Half-Dome 1 used mechanical actuation to move the display. Half-Dome 3 employed an electronic solution using a stack of liquid crystal lenses capable of rapidly switching between 64 discrete focal planes, combined with eye tracking to present the correct focus based on gaze, achieving a wide FoV (~140°).[20]
- CREAL (2020 onwards): This Swiss startup focuses on developing compact lightfield display engines, primarily for AR glasses. Their approach often involves time-multiplexed projection (using sources like micro-LEDs) or scanning combined with holographic combiners to generate many views, aiming for continuous focus cues (for example 0.15m to infinity demonstrated) within a ~50-60° FoV in a form factor suitable for eyeglasses.[21]
Applications
The primary motivation for developing NELFDs is to significantly enhance visual realism, immersion, and comfort in VR and AR applications:
- VR Comfort & Presence: By eliminating the VAC, NELFDs can dramatically reduce eyestrain, fatigue, and nausea during extended VR sessions. The addition of correct focus cues enhances the sense of presence, making virtual objects feel more solid and real, improving depth judgment, and aiding tasks requiring precise spatial awareness or interaction.
- AR Depth Coherence: A critical application where virtual content needs to seamlessly integrate with the real world. NELFDs allow virtual objects to appear at specific, correct optical depths that match real-world objects viewed simultaneously. This is crucial for applications like surgical overlays, industrial assembly guidance (for example projecting instructions onto machinery), architectural previews, and collaborative design visualization.
- Training & Simulation: Applications requiring precise hand-eye coordination (for example flight simulators, driving simulators, medical training simulators for surgery or diagnostics) benefit greatly from accurate rendering of depth and natural focus cues.
- Productivity & Close Work: Enables clear, comfortable viewing of virtual text, user interfaces, or detailed models at close distances within a virtual workspace. This is often problematic and fatiguing in conventional fixed-focus HMDs, limiting their utility for tasks like reading documents or examining intricate virtual objects.
- Entertainment & Gaming: Provides more immersive and visually stunning experiences by adding realistic depth and focus effects.
- Assistive technology: Potential use in vision correction or enhancement beyond simple prescription compensation.
- Telepresence & Communication: More realistic rendering of remote participants or environments could enhance virtual meetings and collaboration.
Current Status and Future Outlook
Near-eye lightfield displays remain predominantly in the research and development phase, although specific implementations like multi-plane displays (for example Magic Leap) and varifocal displays (explored heavily in research like Half-Dome and potentially entering niche products) represent steps in this direction. The significant challenges outlined above, particularly the complex trade-offs between resolution, computational power, field of view, and form factor, have prevented widespread adoption in mainstream consumer HMDs thus far.
Ongoing research and development efforts focus on:
- Novel Display Panels & Optics: Developing higher-resolution, higher-brightness, faster-switching microdisplays (for example microLEDs, advanced OLEDs, fast LCoS) and advanced optical elements (more efficient HOEs, tunable Metasurfaces, improved MLAs potentially using freeform or curved surfaces[2]) to improve the critical spatio-angular resolution trade-off.
- Efficient Computation & Rendering: Creating more efficient algorithms for lightfield rendering (potentially using AI / machine learning for view synthesis, compression, or up-sampling) and dedicated hardware acceleration (ASICs or FPGA designs) to make real-time performance feasible on mobile or wearable platforms.
- Eye Tracking Integration: Leveraging high-speed, high-accuracy eye tracking is becoming crucial. It enables foveated rendering adapted for lightfields (concentrating computational resources and potentially resolution/angular sampling where the user is looking), allows dynamic optimization of the display based on gaze (for example in varifocal systems), potentially relaxes eyebox constraints, and aids calibration.
- Error Correction & Yield Improvement: Exploiting the inherent redundancy in lightfield data (where multiple pixels contribute to the same perceived point from different angles) to computationally correct for manufacturing defects like dead pixels in the microdisplay, potentially improving production yields for large, high-resolution panels.[2]
- Hybrid Approaches: Combining elements of different techniques (for example a small number of switchable focal planes combined with some angular diversity per plane) to achieve a perceptually "good enough" approximation of a true lightfield effect that balances performance and feasibility with current technology.
While significant hurdles remain, continued advances in micro-display technology, computational power (particularly AI-driven methods), optical materials and design (like metasurfaces), and eye-tracking integration hold promise. The long-term goal is to achieve true, continuous lightfield displays delivering imagery optically indistinguishable from reality within lightweight, energy-efficient, eyeglass-sized hardware, which would represent a paradigm shift in personal computing and immersive experiences.
References
- ↑ 1.0 1.1 [Refocus your Eyes: Nvidia presents Near-Eye Light Field Display Prototype | LightField Forum](http://lightfield-forum.com/2013/07/refocus-your-eyes-nvidia-presents-near-eye-light-field-display-prototype/)
- ↑ 2.00 2.01 2.02 2.03 2.04 2.05 2.06 2.07 2.08 2.09 2.10 2.11 Lanman, D., & Luebke, D. (2013). Near-eye light field displays. ACM Transactions on Graphics (TOG), 32(4), Article 138. Presented at SIGGRAPH 2013. PDF Link
- ↑ 3.0 3.1 3.2 Hoffman, D. M., Girshick, A. R., Akeley, K., & Banks, M. S. (2008). Vergence–accommodation conflicts hinder visual performance and cause visual fatigue. Journal of Vision, 8(3), 33. doi:10.1167/8.3.33
- ↑ 4.0 4.1 Stanford Computational Imaging Lab (2015). The Light Field Stereoscope - SIGGGRAPH 2015 [Video]. Retrieved from https://www.youtube.com/watch?v=YJdMPUF8cDM
- ↑ 5.0 5.1 5.2 5.3 Huang, F.‑C., Wetzstein, G., Barsky, B. A., & Heide, F. (2015). The light‑field stereoscope: immersive computer graphics via factored near‑eye light‑field displays with focus cues. ACM Transactions on Graphics, 34(4), Article 60. https://doi.org/10.1145/2766922
- ↑ Bhakta, V.R., Richuso, J. and Jain, A. (2014). DLP ® Technology for Near Eye Display. Texas Instruments White Paper DLPA051. Retrieved from http://www.ti.com/lit/wp/dlpa051/dlpa051.pdf
- ↑ Akşit, K., Chakravarthula, P., Rathinavel, K., Jeong, Y., Albert, R., Fuchs, H., & Luebke, D. (2019). Manufacturing application‑driven foveated near‑eye displays. IEEE Transactions on Visualization and Computer Graphics, 25(5), 1928‑1939. https://doi.org/10.1109/TVCG.2019.2898781
- ↑ Schowengerdt, B. T., & Seibel, E. J. (2015). *True 3‑D scanned voxel displays using single or multiple light sources*. U.S. Patent 9,025,213 B2. Retrieved from https://patents.google.com/patent/US9025213B2
- ↑ 9.0 9.1 9.2 9.3 Maimone, A., Lanman, D., Rathinavel, K., Keller, K., Luebke, D., & Fuchs, H. (2017). Holographic near‑eye displays for virtual and augmented reality. ACM Transactions on Graphics, 36(4), Article 85. https://doi.org/10.1145/3072959.3073624
- ↑ Wetzstein, G., Luebke, D., & Heidrich, W. (2012). Hand‑held computational light‑field photography and display. IEEE Computer Graphics and Applications, 32(1), 8‑13. https://doi.org/10.1109/MCG.2012.15
- ↑ Pamplona, V. F., Oliveira, M. M., Aliaga, D. G., & Raskar, R. (2012). Tailored displays to compensate for visual aberrations. ACM Transactions on Graphics, 31(4), Article 99. https://doi.org/10.1145/2185520.2185577
- ↑ [Naked-eye light field display technology based on mini/micro light emitting diode panels: a systematic review and meta-analysis | Scientific Reports](https://www.nature.com/articles/s41598-024-75172-z)
- ↑ [Frontiers | Challenges and Advancements for AR Optical See-Through Near-Eye Displays: A Review](https://www.frontiersin.org/journals/virtual-reality/articles/10.3389/frvir.2022.838237/full)
- ↑ Lippmann, G. (1908). Épreuves réversibles donnant la sensation du relief [Reversible proofs giving the sensation of relief]. Journal de Physique Théorique et Appliquée, 7(1), 821–825.
- ↑ Steele, B. (2013, July 24). NVIDIA Research’s near‑eye light‑field display prototype eyes‑on (video). Engadget. Retrieved from https://www.engadget.com/2013-07-24-nvidia-research-near-eye-light-field-display-prototype.html
- ↑ Pirzada, U. (2015, August 13). Nvidia Working On Next Generation Virtual Reality Device; The Near Eye Light Field Stereoscope, Aims To Bring It To The Market by 2018. WCCFTech. Retrieved from http://wccftech.com/nvidia-virtual-reality-device-light-field-2018/
- ↑ Worrel, J. (2016, January 30). Nvidia shows off its 'Light Field' VR headset at VRLA 2016. Fudzilla. Retrieved from http://www.fudzilla.com/news/graphics/39762-nvidia-shows-off-its-light-field-vr-headset-at-vrla-2016
- ↑ Avegant (2017, March 16). Avegant Introduces Light Field Technology For Mixed Reality Experiences. PR Newswire. https://www.prnewswire.com/news-releases/avegant-introduces-light-field-technology-for-mixed-reality-300423855.html
- ↑ Hamilton, I. (2018, August 15). Magic Leap One Creator Edition-In‑depth review. UploadVR. Archived at https://web.archive.org/web/20180816062346/https://uploadvr.com/magic-leap-one-review/
- ↑ Abrash, M. (2019, September 25). Oculus Connect 6 Keynote [Video]. YouTube. Retrieved from https://www.youtube.com/watch?v=7YIGT13bdXw (Relevant discussion on Half-Dome prototypes)
- ↑ CREAL (n.d.). Technology. Retrieved from https://creal.com/technology/